The use of intravenous indocyanine green in minimally invasive segmental lung resections: a systematic review
Highlight box
Key findings
• After administration of intravenous indocyanine green (ICG), visualization of the intersegmental plane (ISP) is successful in up to 94% of cases, even after administration of a low dose (0.05 mg/kg) of ICG. The use of intravenous ICG is safe with no reported adverse effects in the immediate peri-operative period.
What is known and what is new?
• Multiple studies have established that the intravenous administration of ICG for delineating the ISP is a secure and viable technique. This systematic review comprehensively examines all findings pertaining to the effectiveness of intravenous ICG in demarcating the intersegmental line.
What is the implication, and what should change now?
• The significance of this systematic review lies in demonstrating that by combining all the available data on intravenous ICG usage, it shows that the intersegmental line can be visualized in 94% of cases.
Introduction
To date, lung cancer is the leading cause of cancer death (1). Since the emergence of a screening program for early detection of lung cancer, there has been an increased incidence of early-stage lung cancer and decreased incidence of advanced-stage lung cancer (2). Until recently, lobectomy was considered as the gold standard for treatment of early-stage non-small cell lung cancer (NSCLC), while sublobar resections were only considered among patients with limited pulmonary function (3-5). However, from a recent meta-analysis by Winckelmans et al. [2020], it became apparent that there are no significant differences in oncological outcomes after segmentectomy compared to lobectomy for stage I NSCLC <2 cm (6). A more recent randomized trial (JCOG0802/WJOG4607L) by Saji et al. [2022] even demonstrated superior overall survival after segmentectomy compared to patients who underwent a lobectomy for small-peripheral NSCLC (i.e., equal to or smaller than 2 cm) (7).
One of the surgical key steps in performing an anatomical segmentectomy is recognition of the intersegmental plane (ISP). A careful division of the ISP is mandatory in terms of functional lung preservation, prevention/avoidance of complications, adequate tumor margins for providing best oncological outcome in terms of disease-free survival and recurrence (8). Conventional methods to delineate the ISP include the inflation-deflation method (9).
In the past decade, more advanced techniques have been developed, which are more suitable for minimally invasive procedures. The procedure involves isolating and dividing the artery of the target segment. Subsequently, indocyanine green (ICG) is injected into a peripheral vein, allowing it to perfuse the entire parenchyma, except for the isolated segment. With the use of a near-infrared camera (NIR), all structures except for the targeted segment will ‘light up’ through fluorescence, allowing delineation of the ISP (10).
The use of ICG has been known for many years but has gained popularity for lung surgery only during the last decade. The initial indications in oncological surgery consisted of sentinel lymph node mapping, intraoperative identification of solid tumors, and angiography during reconstructive surgery (11).
Misaki et al. [2010] was the first to describe the technique in a case series of eight patients. They demonstrated that the target segment was easy to identify and that the use of ICG was safe and feasible and especially useful in minimally invasive techniques and in cases of severe emphysema (12). The recently published expert consensus recommendations of the European Society of Thoracic Surgeons (ESTS) stated that ISP delineation can preferably be performed by systemic ICG (13). The aim of this systematic review is to evaluate the efficacy of intravenous ICG to clearly visualize the ISP while performing video/robot-assisted thoracoscopic segmentectomies. We present this article in accordance with the PRISMA reporting checklist (14) (available at https://tlcr.amegroups.com/article/view/10.21037/tlcr-23-807/rc).
Methods
Prior to initiation, a review protocol was drafted and submitted to the PROSPERO registry (CRD42022328771).
Eligibility criteria
Types of participants
All patients who underwent a video/robot-assisted thoracoscopic invasive segmentectomy of the lung were considered for inclusion.
Types of interventions
All studies evaluating patients, who underwent a segmentectomy of the lung through uniportal or multiportal video-assisted thoracoscopic surgery (uVATS or mVATS respectively) and/or robot-assisted thoracoscopic surgery (RATS), using intravenous ICG to visualize the ISP were considered. Alternative techniques and interventions, such as endobronchial ICG were excluded.
Outcome measures
The primary outcome was the frequency and percentage of patients in whom the ISP was adequately visualized with the use of intravenous ICG such that a segmentectomy could be performed, including calculation of a weighted mean. Secondary outcomes were ICG dose, time to visualization, time to maximum ICG visualization, time to disappearance of ICG effect and the adverse reactions to ICG. Studies not reporting on the primary outcome were excluded.
Types of studies
Case reports were excluded. In the case of overlapping patient cohorts being described by different studies, the study describing the largest sample size was evaluated. Studies evaluating less than 10 participants were also excluded.
Search and study selection
Potentially eligible papers were identified by searching the electronic PubMed, Embase, Web of Science, the Cochrane Library and CINAHL databases. In addition, the PROSPERO, WHO-ICTRP, and ClinicalTrials.gov registries were systematically searched. Details are listed in supplementary materials. A manual cross-reference and related article search were conducted to identify articles that were not found through the prior search. Articles published in English, French and Dutch were evaluated for inclusion. No publication date restrictions were imposed. All searches were performed by a trained researcher. The last search was run on November 19th, 2021. Studies were screened for eligibility based on their title and abstract. Subsequently, full texts of potentially eligible reports were comprehensively assessed according to the predefined eligibility criteria. Studies adhering to these criteria were included for review and if possible, for meta-analysis. Studies in Dutch, English and French were included. Two independent reviewers (M.P., Y.J.) performed the process of study selection in a nonblinded standardized manner. Potential inter-reviewer disagreements were resolved by consultation of the senior author (E.R.d.L.).
Data collection
Data were extracted by one independent reviewer (M.P.), while its correctness was validated by a second reviewer (Y.J.). Inter-reviewer disagreements were, as foregoing, resolved by consultation of the senior author (E.R.d.L.). Studies reporting continuous variables as mean and standard deviation (SD) were extracted as such, while those reported in any other way were first converted applying the methods by Wan et al. (15). For studies reporting individual patient data, the mean and SD were calculated. Data was extracted from each included paper on: (I) general study characteristics: study design, institution, country and enrollment period; (II) characteristics of participants: number of included participants, sex, and age; (III) characteristics of the procedure: surgical technique (mVATS, uVATS or RATS); (IV) characteristics of the ICG usage: dose, number of uses; time until ICG becomes visible (in seconds); time until maximum effect (in seconds); time until ICG totally disappeared (in seconds), frequency and percentage of patients in whom the ISP is adequately visualized, adverse reaction to ICG.
Risk of bias across studies
Randomized controlled trials were evaluated by the 5-point Jadad scale that assesses randomization (2 points), blinding (2 points) and accountability (1 point). Randomized controlled trials that scored 3–5 points were of high methodological quality (16). The risk of bias in non-randomized studies was assessed by the MINORS (methodological index for non-randomized studies) criteria. For the MINORS criteria, eight items were scored for non-comparative studies and 12 items for comparative studies, with a maximum of 2 points to be awarded per item. The maximum item score is 2, the ideal global score would be 16 for the non-comparative studies and 24 for comparative studies (17). All articles were scored by two reviewers (M.P. and Y.J.). Inter-reviewer disagreements were resolved by consultation of the senior author (E.R.d.L.).
Results
Study selection
A cumulative number of 640 records were retrieved through PubMed (n=221), Embase (n=351), Cochrane Library (n=31), CINAHL (n=29), and Web of Science (n=8) (Figure 1). After removing duplicates (n=155) and screening of the articles’ titles and abstracts, the full text of 105 studies were evaluated. Eventually, 18 articles met the predefined inclusion criteria. The reasons for exclusion were the use of other techniques for the visualization of ISPs including endobronchial ICG use (n=37), alternative study design including case reports (n=30) and review articles (n=9) or articles in a foreign language (n=11).
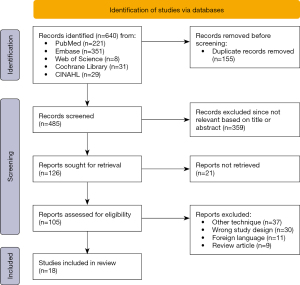
Study characteristics
Study methods
Most of the papers (14/18) described single-center studies (18-31) and 11 studies were retrospective cohort studies (18,20-22,25-30,32). The studies of Misaki et al. [2020] (33), Liu et al. [2020] (28) and Sun et al. [2021] (27) were comparative trials comparing: bolus versus continuous administration of ICG, ICG versus inflation-deflation method or ICG versus modified inflation-deflation method respectively. No randomized controlled trials were identified. All studies were published between 2014 and 2021 while they enrolled patients between October 2008 and August 2020. A detailed description of the included studies is found in Table 1.
Table 1
References | Year of publication | Single or multi center | Start enrollment period | End enrollment period | Institution | Type of study |
---|---|---|---|---|---|---|
Tarumi et al. (18) | 2014 | Single | Oct-08 | Sep-11 | Kagawa University, Japan | Retrospective cohort |
Iizuka et al. (34) | 2016 | Multi | Jun-13 | Apr-15 | Aichi Cancer Center Hospital and Innovative Clinical Research Center (Kanazawa University), Japan | Prospective cohort |
Pischik et al. (20) | 2018 | Single | Sep-15 | Dec-17 | Saint Petersburg State University, Russia | Retrospective cohort |
Bédat et al. (21) | 2018 | Single | Nov-14 | Nov-17 | University Hospital of Geneva, Switzerland | Retrospective cohort |
Kuroda et al. (19) | 2018 | Single | Mar-15 | Mar-16 | Aichi Cancer Center Hospital, Japan | Prospective cohort |
Mehta et al. (35) | 2019 | Multi | Sep-16 | May-18 | McMaster University, Canada; University of Toronto, Canada | Prospective cohort |
Matsuura et al. (22) | 2019 | Single | Jan-13 | Dec-17 | Cancer Institute Hospital of Japanese Foundation for Cancer research, Japan | Retrospective cohort |
Chen et al. (23) | 2019 | Single | Jul-17 | Dec-17 | General Hospital of PLA, China | Prospective cohort |
Zhang et al. (24) | 2019 | Single | Jan-18 | Apr-18 | Guandong General Hospital. The Second Affiliated Hospital of Guangzhou University of Chinese Medicine, Guangzhou | Prospective cohort |
Jin et al. (25) | 2019 | Single | Apr-18 | Oct-18 | Changzheng Hospital, China | Retrospective cohort |
Sun et al. (26) | 2019 | Single | Mar-19 | Jul-19 | Nanjing Medical University, China | Retrospective cohort |
Yajima et al. (30) | 2019 | Single | Sep-18 | Dec-18 | Gunma University Hospital, Japan | Retrospective cohort |
Kim et al. (32) | 2020 | Multi | Mar-16 | Jul-19 | Georgia Institute of Technology, USA; Korea University Guro Hospital, Korea University college of Medicine, Korea | Retrospective cohort |
Misaki et al. (33) | 2020 | Multi | Apr-17 | Aug-19 | Takamatsu Muncipal Hospital, Japan | Prospective comparative |
Liu et al. (28) | 2020 | Single | Sep-17 | Aug-19 | Nanjing Medical University, China | Retrospective comparative |
Matsui et al. (31) | 2021 | Single | Oct-15 | Oct-17 | Aichi Cancer Center Hospital, Japan | Prospective cohort |
Yotsukura et al. (29) | 2021 | Single | Sep-18 | Dec-19 | Nation Cancer Hospital, Japan | Retrospective cohort |
Sun et al. (27) | 2021 | Single | Feb-18 | Aug-20 | Nanjing Medical University, China | Retrospective comparative |
Participants and intervention
A total of 1,090 unique patients underwent 1,094 surgical procedures for 1,097 sublobar anatomic resections with the use of intravenous ICG. In the study of Pischik et al. [2018], 86 patients underwent 90 segmentectomies; one patient underwent two consecutive segmentectomies for bilateral arteriovenous malformations. Three other patients required re-operation for a new solitary lung metastasis (20). In the study of Iizuka et al. [2016], 71 patients underwent segmentectomy of total of 74 segments or subsegments without specifying for what reason they may have undergone multiple (sub)segment resections (34). Study and participant characteristics are illustrated in Table 2. Mean age of the groups ranged from 50.6 to 75.4 years. The number of male patients ranged from 32% to 70%. The most common minimally invasive technique was mVATS (n=13 studies) (18-25,29-31,33,34) (Table 2).
Table 2
References | No. of surgical procedures | Operation | Male, n (%) | Age, years | Dose ICG | No. of injections | Mean time (s) | Mean total time (s) | ISP visible | AE |
---|---|---|---|---|---|---|---|---|---|---|
Tarumi et al. (18) | 13 | mVATS | 6 (46%) | 70.2±2.6 | 3 mg/kg | 1 | NR | NR | 84.6% | NR |
Iizuka et al. (34) | 71* | mVATS | 34 (48%) | 66.4±10.8 | 5 mg | NR | NR | NR | 98.6% | NR |
Pischik et al. (20) | 90** | mVATS | 34 (40%) | 55.2±18.1 | 0.15 mg/kg | 1 | 10 to 25 | NR | 95.6% | 0 |
Bédat et al. (21) | 67 | mVATS | 28 (42%) | 66±10 | 12.5 mg | 1 | NR | NR | 88.0% | 0 |
Kuroda et al. (19) | 29 | mVATS | 16 (55%) | 70±10.9 | 0.05–0.1 mg/kg | NR | NR | NR | 100.0% | NR |
Mehta et al. (35) | 31 | RATS | 17 (55%) | 67.6±8.3 | 15–20 mg | 1 | NR | NR | 100.0% | 0 |
Matsuura et al. (22) | 149 | mVATS | 75 (50%) | 65.4±10.8 | 0.25 mg/kg | 1 | NR | NR | 98.0% | 0 |
Chen et al. (23) | 19 | mVATS | 9 (47%) | 56.4±4.9 | 25 mg | 1 (1×2) | NR | NR | 100.0% | 0 |
Zhang et al. (24) | 11 | mVATS | 5 (45%) | 56±11.61 | 0.25–0.5 mg | 1 | NR | NR | 100.0% | NR |
Jin et al. (25) | 21 | mVATS | 12 (57%) | 53.7±8.4 | 0.5 mg/kg | 1 | NR | NR | 100.0% | 0 |
Sun et al. (26) | 19 | uVATS | 6 (32%) | 50.6±13.7 | 5 mg | 1 | NR | NR | 100.0% | 0 |
Yajima et al. (30) | 16 | mVATS | 11 (69%) | 71.6±5.4 | 0.5 mg/kg | NR | NR | NR | 88% | 0 |
Kim et al. (32) | 31 | 22 uVATS; 9 RATS |
17 (55%) | 63.2±9.8 | 0.3–0.5 mg/kg | 1 | NR | NR | 100.0% | NR |
Misaki et al. (33) | 10 | mVATS | 7 (70%) | 72±11.2 | 0.09±0.009 mg/kg | 1 | 36±13 | 170±23 | 30.0% | NR |
10 | mVATS | 7 (70%) | 75.4±10.3 | 0.11±0.04 mg/kg, 300 mL/h, max 0.3 mg/kg |
Constant | 40±29 | 140±54 | 90.0% | NR | |
Liu et al. (28) | 92 | uVATS | 43 (47%) | 69.8±5.2 | 15–20 mg | 1 | 15 | NR | 91.4% | NR |
Matsui et al. (31) | 106 | mVATS | 51 (48%) | 67.4±9.3 | 0.05–0.1 mg/kg | NR | NR | NR | 100% | 0 |
Yotsukura et al. (29) | 209 | mVATS | 87 (42%) | 67.9±11.6 | 0.25 mg/kg | 1 | 16±10 | 99±77 | 88.0% | 0 |
Sun et al. (27) | 100 | uVATS | 43 (43%) | 59.8±10.5 | 5 mg (max 25 mg) | 1 or more | 24±4 | NR | 98.0% | 0 |
*, in the study of Iizuka et al. [2016], 71 patients underwent 74 segments or subsegments; **, in the study of Pischik et al. [2018], 86 patients underwent 90 segmentectomies. Mean time: mean time before clearly visible, presented as mean ± standard deviation or range. Mean total time: mean total time ICG is still visible, presented as mean ± standard deviation. ICG, indocyanine green; ISP, intersegmental plane; AE, adverse events; mVATS, multiportal video-assisted thoracoscopic surgery; NR, not reported; RATS, robot-assisted thoracoscopic surgery; uVATS, uniportal video-assisted thoracoscopic surgery.
Outcome
The percentage of ISP visualization per study is provided in Table 2. The weighted mean of ISP visualization was 94% and ranged from 30% to 100%. The dose of ICG administered was unique and greatly differing for almost all studies (Table 2). Several (n=8) used fixed doses while others systematically used a weight-based dose (n=10). A bolus of ICG was administered in all but one study, in which a subgroup of patients received a continuous perfusion of ICG. In eight of the eighteen studies, the ISP was visible after a bolus injection in 100% of those cases (19,23-26,31,32,35). Twelve studies reported that only a single shot ICG was administered (18,20-22,24-26,28,29,32,33,35), and four studies did not report whether one or multiple doses were given (19,30,31,34).
Two studies explored the use of an additional ICG bolus to enhance ISP visibility. In the study of Chen et al. [2019], one patient received a second dose of ICG because the ISP was not adequately visible. After the second dose this was clearly visible (23). In the study of Sun et al. [2021] it was reported that some patients received multiple doses but did not specify the total number of patients, nor the effect on ISP visibility (27).
The study of Misaki et al. [2020] compared a bolus injection (10 patients with a bolus of ICG of 0.09 mg/kg) to a constant infusion (10 patients at constant infusion rate with a mean dose of 0.11 mg/kg of 300 mL/h with a maximum of 0.3 mg/kg). They concluded that the ISP was identified in more cases following a constant infusion rate versus a bolus injection with an ISP identification of 90% and 30% respectively (P=0.0003) (33).
Several studies explored additional techniques to enhance visibility. In the study of Yajima et al. [2019], a group of 16 patients underwent an upper lobe segmentectomy. Nine patients underwent segmentectomy with identification of the intersegmental border by using the interlobar pulmonary artery compression method. In this method, the interlobar pulmonary artery is compressed while ICG is administered intravenous so that there is an increase in blood supply to the upper lobe. In all cases (n=9/9) the intersegmental line was clearly visible. The other 7 patients underwent segmentectomy with the conventional method. In two cases the intersegmental line was not visible; in two cases the intersegmental line was only partially visible (n=2/7) (30).
Eleven studies reported on potential adverse effects of ICG. Regardless of the ICG dose, no adverse reactions were reported in these studies (20-23,25-27,29-31,35). The mean time before the first effect of the ICG administration was visible ranged from 10 to 40 seconds. The mean total time of the ICG visibility ranged from 90 to 140 seconds after a bolus injection and was 170 seconds after a constant infusion rate (20,27-29,33).
Risk of bias across studies
All articles are scored following the MINORS criteria (Table S1). There were 15 non-comparative studies and 3 comparative studies. The minor score for the non-comparative study scored low to moderate with scores ranging from 4 to 11 of a maximum of 16 (19-26,29-32,34,35). The comparative studies all scored moderate, ranging from 14 to 18 of a maximum of 24 (27,28,33). In most studies, the main limiting factors were the fact that study endpoint was not clear before start of the study and that there was lacking information on loss to follow-up.
Discussion
This systematic review aimed to evaluate the efficacy of intravenous ICG to clearly visualize the ISP while performing segmentectomies. The weighted mean for ISP visualization was 94% of the cases (range, 30–100%). This review revealed notable diversity in ICG administration protocols between studies, with variable fixed or weight-based doses applied once or multiple times, or even by continuous perfusion. Despite these variations, no related adverse events were reported, revealing the safety of ICG for this purpose. The range of ISP visualization was highly discordant in this review due to the lowermost value of 30% in a patient arm of the study by Misaki et al. [2020]. All other studies had an ISP visualization score of 84.6% or higher. The work of Misaki et al. [2020] provided valuable insights since they compared the impact of bolus injection to continuous infusion on ISP identification. They reported a higher identification rate (90%) and fluorescence intensity following continuous infusion compared to a bolus injection (30%), highlighting the potential benefits of this alternative administration techniques. In contrast to a bolus injection, administering ICG at a constant rate ensures the steady and appropriate maintenance of blood concentrations. It is important to note that for this comparative study a small patient group was used with 10 patients in each arm (bolus vs. constant infusion) (33).
The administered dose between studies ranged from a fixed dose of 5 to 25 mg and weight-based dose of 0.05 to 3 mg/kg. This variable weight-based dose will, for instance in a patient weighing 70 kg, result in an ICG bolus of 3.5 to 210 mg, respectively. In eight studies, the ISP visualization was clearly visible in 100% of the cases. In these eight studies, both weight-based dose (0.05 to 0.5 mg/kg) as fixed dose (5 to 25 mg) was used. Contra-intuitive, in the study with the highest dose, 3 mg/kg, the ISP was visible in only 84.6% of the cases. Therefore, one may question whether an optimal dosage can effectively be advised given the large heterogeneity of results with generally good ISP visualization. This suggests that other factors than dose influence the efficacy of ICG. Moreover, the study of Misaki et al. [2020] applied 0.09 mg/kg of bolus ICG in one study arm, which is comparable to the dose of 0.05–0.1 mg/kg used in the study of Matsui et al. [2021], though both studies reported different outcome, with 30% and 100%, respectively (31,33).
Repeated doses of ICG (respectively 25 and 5 mg) until ISP was shown clearly, were used in the trials of Chen et al. [2019] and Sun et al. [2021], leading to adequate ISP identifications in 98% and 100% respectively (23,27). Given the limited data on the effect of administration of multiple doses of ICG, no conclusions can be drawn on the usefulness of repetitive doses to improve visualization of the ISP. Furthermore, several other variables could influence the formation of an adequate ISP. Iizuka et al. [2016] demonstrated that a Smoking Index (SI) >800 and low attenuation area (LAA) on computed tomography >1%. were correlated with a worse visibility since the tissue density of emphysematous and bullous lung tissues is lower than that of normal lung tissue. They also reported that ICG visualization was challenging in individuals with extensive anthracosis in contrast to those with mild-to-moderate anthracosis, although this observation was drawn from expert opinions. Examination of a lung specimen from a smoker under transmitted light revealed increased brightness only around a bronchiole, indicating reduced light permeability within intrapulmonary structures affected by anthracosis (34). This could be an argument for the low visibility in the trial by Misaki et al. [2020] with adequate ISP visualization in only 30% of the cases (n=10). It is important to realize that in the other remaining cases, the ISP was judged as thin, mottled or badly visible due to and early washed-out of the ICG. Furthermore, the population in this trial contained the highest percentage of smokers and the lowest percentage of forced expiratory volume in the first second (FEV1). In emphysematous lungs, which are less perfused, the ISP could be less visible or the identified ISP misleading or confusing following ICG injection (33). Therefore, taking all factors into account, we cannot confidently state that administering ICG through continuous infusion reliably improves the visualization of the ISP.
A noteworthy secondary outcome remains to the safety of ICG administration. Eleven of the eighteen studies reported that there were no adverse reactions to ICG. Speich et al. [1988] showed in a prior study that the frequency of allergic reactions with ICG doses below 0.5 mg/kg was reported at 0.003%, but significantly increased if the dose exceeded 5 mg/kg (36). In none of the included trials, the doses exceeded 5 mg/kg.
An alternative approach employing ICG for ISP visualization involves the endobronchial administration of the dye. In this technique, diluted ICG is introduced into each intended target segmental or subsegmental bronchus. This method offers the advantage of not requiring lung inflation, which conserves space during minimally invasive thoracoscopic surgeries. However, a drawback of this approach is the potential for the dye to retrograde from the target bronchus, spreading within the bronchial tree and potentially compromising ISP identification. Moreover, the presence of a bronchoscopy-experienced medical professional is essential for this technique’s successful execution (8,37). No trials comparing intravenous ICG to endobronchial ICG were identified.
An alternative method for the identification of ISP is the inflation-deflation technique where respectively the target segment is inflated or deflated during the procedure. This technique has the advantage of requiring no specialized preoperative preparations and is the most straightforward to execute during surgical intervention. Nevertheless, it presents certain limitations, including impaired surgical visualization, particularly evident in minimally invasive procedures, and potential complications related to collateral ventilation, particularly when the target bronchus has been previously ligated or excised (9). When looking at the outcome of inflation and deflation compared to ICG, the retrospective study of Sun et al. [2021] already highlighted that time to ISP visualization was significantly faster after ICG injection than after the use of the inflation-deflation method with visualization time respectively of 23.59 (SD 4.47) vs. 1,026.80 (SD 318.34) seconds (P<0.001) (27). Thus, based on this data, ICG provides fast yet only short-term visualization of the ISP. In the reviewed literature there were two retrospective cohort studies, and both indicated a better development of intersegmental lines, shorter operation time and less prolonged air leaks when ICG was used compared to the inflation-deflation method (27,28). So the use of ICG, in comparison to deflation-inflation, is more user-friendly and with a faster visualization of the ISP without impacting the working space.
Furthermore, hardly any side effects have been described in literature and the dose can be repeated in case of reduced visualization. In the consensus-based guideline from the ESTS in 2023, they recommended defining the ISPs preferably by using near-infrared imaging (NIRI) after systemic injection of ICG (13). The only drawback of this technique, however, is that an infrared camera must be available, and this may cause a considerable additional cost. However, based on these findings, one could speculate that the use of ICG may also potentially result in cost savings. The use of ICG could shorten operation time, leading to cost savings in terms of surgical planning. Additionally, ICG usage might contribute to reduced occurrences of prolonged air leakage, presenting another potential option for cost savings. However, it is essential to note that these considerations are currently hypothetical, and there is insufficient empirical evidence to definitively establish the cost-effectiveness of ICG in these aspects. So, at this moment based on the published data, it is not possible to make an adequate cost-benefit analysis. One of the major limitations of this systematic review is that the discussed studies were performed in small cohorts of patients. No randomized controlled trials were identified. Overall, it is apparent that there is a considerable heterogeneity regarding the injected dose of ICG and the reported outcome measures. In general, the quality of all the published evidence is low.
Perhaps the most important limitation is that the primary endpoint, adequate visualization of the ISP, is not a clearly objectively measurable primary endpoint. The visualization is obviously subjective and is co-determined by the surgeon’s interpretation, the NIR camera used, the darkening in the operating theatre, etc. In the future, dose-escalation studies may be interesting to determine the most appropriate dose at which a maximum effect can be obtained. Further, a randomized controlled trials comparing the inflation-deflation technique or intrabronchial ICG to intravenous ICG, to identify differences in ISP identification efficacy, clinical outcome, and cost might be interesting.
Conclusions
The use of intravenous ICG is a safe and effective technique, demonstrating clear ISP visualization in up to 94% of cases. While there is variability in dosing strategies, even low doses (0.05 mg/kg) can achieve good visualization. It is considered safe to administer ICG once or multiple times, as supported by the literature reporting minimal side effects. Currently, ICG stands out as a preferred technique for ISP visualization based on the available guidelines.
Acknowledgments
Funding: None.
Footnote
Reporting Checklist: The authors have completed the PRISMA reporting checklist. Available at https://tlcr.amegroups.com/article/view/10.21037/tlcr-23-807/rc
Peer Review File: Available at https://tlcr.amegroups.com/article/view/10.21037/tlcr-23-807/prf
Conflicts of Interest: All authors have completed the ICMJE uniform disclosure form (available at https://tlcr.amegroups.com/article/view/10.21037/tlcr-23-807/coif). The authors have no conflicts of interest to declare.
Ethical Statement: The authors are accountable for all aspects of the work in ensuring that questions related to the accuracy or integrity of any part of the work are appropriately investigated and resolved.
Open Access Statement: This is an Open Access article distributed in accordance with the Creative Commons Attribution-NonCommercial-NoDerivs 4.0 International License (CC BY-NC-ND 4.0), which permits the non-commercial replication and distribution of the article with the strict proviso that no changes or edits are made and the original work is properly cited (including links to both the formal publication through the relevant DOI and the license). See: https://creativecommons.org/licenses/by-nc-nd/4.0/.
References
- ECIS – European Cancer Information System. 2020 new cases (incidence) and deaths (mortality) estimates Lung cancer burden in EU-27. Available online: https://ecis.jrc.ec.europa.eu/pdf/factsheets/Lung_cancer_en-July_2021.pdf
- Khouzam MS, Wood DE, Vigneswaran W, et al. Impact of Federal Lung Cancer Screening Policy on the Incidence of Early-stage Lung Cancer. Ann Thorac Surg 2023;115:827-33. [Crossref] [PubMed]
- Kilic A, Schuchert MJ, Pettiford BL, et al. Anatomic segmentectomy for stage I non-small cell lung cancer in the elderly. Ann Thorac Surg 2009;87:1662-6; discussion 1667-8. [Crossref] [PubMed]
- Tsutani Y, Tsubokawa N, Ito M, et al. Postoperative complications and prognosis after lobar resection versus sublobar resection in elderly patients with clinical Stage I non-small-cell lung cancer. Eur J Cardiothorac Surg 2018;53:366-71. [Crossref] [PubMed]
- Miller JI, Hatcher CR Jr. Limited resection of bronchogenic carcinoma in the patient with marked impairment of pulmonary function. Ann Thorac Surg 1987;44:340-3. [Crossref] [PubMed]
- Winckelmans T, Decaluwé H, De Leyn P, et al. Segmentectomy or lobectomy for early-stage non-small-cell lung cancer: a systematic review and meta-analysis. Eur J Cardiothorac Surg 2020;57:1051-60. [Crossref] [PubMed]
- Saji H, Okada M, Tsuboi M, et al. Segmentectomy versus lobectomy in small-sized peripheral non-small-cell lung cancer (JCOG0802/WJOG4607L): a multicentre, open-label, phase 3, randomised, controlled, non-inferiority trial. Lancet 2022;399:1607-17. [Crossref] [PubMed]
- Nex G, Schiavone M, De Palma A, et al. How to identify intersegmental planes in performing sublobar anatomical resections. J Thorac Dis 2020;12:3369-75. [Crossref] [PubMed]
- Sato M, Murayama T, Nakajima J. Concepts and techniques: how to determine and identify the appropriate target segment in anatomical pulmonary segmentectomy? J Thorac Dis 2019;11:972-86. [Crossref] [PubMed]
- Andolfi M, Potenza R, Seguin-Givelet A, et al. Identification of the intersegmental plane during thoracoscopic segmentectomy: state of the art. Interact Cardiovasc Thorac Surg 2020;30:329-36. [Crossref] [PubMed]
- Schaafsma BE, Mieog JS, Hutteman M, et al. The clinical use of indocyanine green as a near-infrared fluorescent contrast agent for image-guided oncologic surgery. J Surg Oncol 2011;104:323-32. [Crossref] [PubMed]
- Misaki N, Chang SS, Igai H, et al. New clinically applicable method for visualizing adjacent lung segments using an infrared thoracoscopy system. J Thorac Cardiovasc Surg 2010;140:752-6. [Crossref] [PubMed]
- Brunelli A, Decaluwe H, Gonzalez M, et al. European Society of Thoracic Surgeons expert consensus recommendations on technical standards of segmentectomy for primary lung cancer. Eur J Cardiothorac Surg 2023;63:ezad224. [Crossref] [PubMed]
- Page MJ, McKenzie JE, Bossuyt PM, et al. The PRISMA 2020 statement: an updated guideline for reporting systematic reviews. BMJ 2021;372: [PubMed]
- Wan X, Wang W, Liu J, et al. Estimating the sample mean and standard deviation from the sample size, median, range and/or interquartile range. BMC Med Res Methodol 2014;14:135. [Crossref] [PubMed]
- Jadad AR, Moore RA, Carroll D, et al. Assessing the quality of reports of randomized clinical trials: is blinding necessary? Control Clin Trials 1996;17:1-12. [Crossref] [PubMed]
- Slim K, Nini E, Forestier D, et al. Methodological index for non-randomized studies (minors): development and validation of a new instrument. ANZ J Surg 2003;73:712-6. [Crossref] [PubMed]
- Tarumi S, Misaki N, Kasai Y, et al. Clinical trial of video-assisted thoracoscopic segmentectomy using infrared thoracoscopy with indocyanine green. Eur J Cardiothorac Surg 2014;46:112-5. [Crossref] [PubMed]
- Kuroda H, Yoshida T, Arimura T, et al. Novel development of Spectra-A using indocyanine green for segmental boundary visibility in thoracoscopic segmentectomy. J Surg Res 2018;227:228-33. [Crossref] [PubMed]
- Pischik VG, Kovalenko A. The role of indocyanine green fluorescence for intersegmental plane identification during video-assisted thoracoscopic surgery segmentectomies. J Thorac Dis 2018;10:S3704-11. [Crossref] [PubMed]
- Bédat B, Triponez F, Sadowski SM, et al. Impact of near-infrared angiography on the quality of anatomical resection during video-assisted thoracic surgery segmentectomy. J Thorac Dis 2018;10:S1229-34. [Crossref] [PubMed]
- Matsuura Y, Mun M, Ichinose J, et al. Recent fluorescence-based optical imaging for video-assisted thoracoscopic surgery segmentectomy. Ann Transl Med 2019;7:32. [Crossref] [PubMed]
- Chen R, Ma Y, Li C, et al. A Pilot Study of Pulmonary Segmentectomy With Indocyanine Green Near-Infrared Angiography. Surg Innov 2019;26:337-43. [Crossref] [PubMed]
- Zhang C, Lin H, Fu R, et al. Application of indocyanine green fluorescence for precision sublobar resection. Thorac Cancer 2019;10:624-30. [Crossref] [PubMed]
- Jin Y, Wang M, Xue L, et al. Clinical Application of Near-Infrared Thoracoscopy With Indocyanine Green in Video-Assisted Thoracoscopic Anatomical Segmentectomy. Surg Innov 2019;26:473-7. [Crossref] [PubMed]
- Sun Y, Zhang Q, Wang Z, et al. Is the near-infrared fluorescence imaging with intravenous indocyanine green method for identifying the intersegmental plane concordant with the modified inflation-deflation method in lung segmentectomy? Thorac Cancer 2019;10:2013-21. [Crossref] [PubMed]
- Sun Y, Zhang Q, Wang Z, et al. Feasibility investigation of near-infrared fluorescence imaging with intravenous indocyanine green method in uniport video-assisted thoracoscopic anatomical segmentectomy for identifying the intersegmental boundary line. Thorac Cancer 2021;12:1407-14. [Crossref] [PubMed]
- Liu Z, Yang R, Cao H. Near-infrared intraoperative imaging with indocyanine green is beneficial in video-assisted thoracoscopic segmentectomy for patients with chronic lung diseases: a retrospective single-center propensity-score matched analysis. J Cardiothorac Surg 2020;15:303. [Crossref] [PubMed]
- Yotsukura M, Okubo Y, Yoshida Y, et al. Indocyanine green imaging for pulmonary segmentectomy. JTCVS Tech 2021;6:151-8. [Crossref] [PubMed]
- Yajima T, Shimizu K, Mogi A, et al. Pulmonary Artery Compression Facilitates Intersegmental Border Visualization. Ann Thorac Surg 2019;108:e141-3. [Crossref] [PubMed]
- Matsui T, Takahashi Y, Nakada T, et al. Efficacy of Xenon Light With Indocyanine Green for Intersegmental Visibility in Thoracoscopic Segmentectomy. J Surg Res 2021;259:39-46. [Crossref] [PubMed]
- Kim Y, Rho J, Quan YH, et al. Simultaneous visualization of pulmonary nodules and intersegmental planes on fluorescent images in pulmonary segmentectomy. Eur J Cardiothorac Surg 2020;58:i77-84. [Crossref] [PubMed]
- Misaki N, Tatakawa K, Chang SS, et al. Constant-rate intravenous infusion of indocyanine green leading to high fluorescence intensity in infrared thoracoscopic segmentectomy. JTCVS Tech 2020;3:319-24. [Crossref] [PubMed]
- Iizuka S, Kuroda H, Yoshimura K, et al. Predictors of indocyanine green visualization during fluorescence imaging for segmental plane formation in thoracoscopic anatomical segmentectomy. J Thorac Dis 2016;8:985-91. [Crossref] [PubMed]
- Mehta M, Patel YS, Yasufuku K, et al. Near-infrared mapping with indocyanine green is associated with an increase in oncological margin length in minimally invasive segmentectomy. J Thorac Cardiovasc Surg 2019;157:2029-35. [Crossref] [PubMed]
- Speich R, Saesseli B, Hoffmann U, et al. Anaphylactoid reactions after indocyanine-green administration. Ann Intern Med 1988;109:345-6. [Crossref] [PubMed]
- Sekine Y, Ko E, Oishi H, et al. A simple and effective technique for identification of intersegmental planes by infrared thoracoscopy after transbronchial injection of indocyanine green. J Thorac Cardiovasc Surg 2012;143:1330-5. [Crossref] [PubMed]